Concordes Vice President of Advanced Battery Technology, on Sun Xtender® Renewable Energy Batteries: including maximizing the life of your storage battery & battery aging, technology costs and risks
Battery Technology from A to Z
David Vutetakis | Sun Xtender
How long should my Sun Xtender® batteries last?
This is one of the hardest questions to answer because battery life depends on so many factors. The short answer is that the battery lifespan can range anywhere from 1 to 10+ years, depending on how the batteries are operated. To get more specific, let us consider two common scenarios:
(a) The first scenario is an off‐grid system with a daily discharge/charge cycle. If the capacity removed from the battery is fairly consistent day after day, then the total number of cycles can be estimated from the cycle life vs DOD chart in the Technical Manual. For example, if the average DOD is 50%, then the estimated cycle life is about 1000 cycles, or 1000/365 = 2.7 years. Likewise, if the average DOD is 25%, then the estimated cycle life is approximately 2500 cycle, or 2500/365 = 6.8 years. It should be recognized that these numbers are based on carefully controlled laboratory conditions, so the actual cycle life for an installed bank of batteries may be less.
(b) The second scenario is a grid‐tied system with a battery being used as a back‐up in the event of a power outage. In this scenario the battery spends most of its time on float charge and is rarely called upon to provide a discharge cycle. The float life of Sun Xtender® batteries is strongly dependent on the temperature environment. If the battery bank is consistently maintained at 20°C, a float life of approximately 6‐10+ years is achievable. However, at higher temperatures, the float life will be significantly lower. As a rule of thumb, the float life will be cut in half for every 10°C increase in temperature above 20°C. For example, a battery bank that lasts 8 years at 20°C would last only 4 years at 30°C and only 2 years at 40°C. Therefore, to maximize battery life, it is a good idea to put the battery bank in temperature environment that is maintained as close to 20°C as possible.
What value should I use for my low voltage disconnect (LVD) setpoint?
This question is a common one and there are several factors that need to be considered. As the term implies, the LVD is the voltage at which the battery is disconnected from the load and an alternate power source is switched on, such as a generator. The LVD protects the battery from being over‐discharged, which can rapidly damage the battery and lead to premature failure. To provide specific recommendations, there are three common scenarios to consider.
(a) In the first scenario, the DOD is 50% or less per day and is fairly consistent day after day. In this scenario, the battery only needs protection from being over‐discharged, which could occur due to a system problem (for example, the charge controller stops working). The LVD voltage in this scenario should be set to correspond to an 80% DOD. The voltage at 80% DOD depends on the load, and there is a table in the Technical Manual that provide the voltage vs load data. For example, with a load that corresponds to an 8‐hour discharge rate, the voltage at 80% DOD is 11.6 volts. Thus, the LVD should be set at 11.6 volts (for a 12V bank, multiply by 2 for a 24‐volt bank and by 4 for a 48‐volt bank).
(b) In the second scenario, the battery is being used as a back‐up in a grid‐tied system. In the event of an extended power outage, the battery should be protected from being over‐discharged. Once the LVD is reached, all loads will be switched off unless there is another power source available such as a generator. The setpoint in this scenario should normally be the same as in scenario (a), corresponding to 80% DOD. However, if it is imperative to power loads as long as possible during an extended power outage, the LVD could be set to 100% DOD because this condition would occur infrequently and the batteries will be fully recharged once power is restored.
(c) In the third scenario, the battery experiences a variable DOD that can sometimes exceed 50% on heavy use days. In this scenario, the LVD can be used as a trigger to turn on the generator once the battery reaches 50% DOD. It should also be noted that for all three scenarios, if the load on the battery has peaks that are significantly higher than the base load (such as a pump motor), the voltage will dip during these peaks and could miscue the LVD. Most LVD manufacturers get around this by building a time delay into the circuit (typically 20 seconds to 2 minutes) so it will ride through the peaks.
Why is my generator running more frequently this year compared with previous years?
To answer this question, we must explain what happens when a battery ages. The capacity of a new battery bank will be close to 100% of its nameplate rating. For example, the PVX‐3050T is a 6‐volt battery rated at 305 Ampere‐hours (Ah) at the 24‐hour rate. If a 48‐volt bank of these batteries is discharged at 305Ah/24h = 12.7A, it will run about 24 hours to the endpoint voltage of 42 volts (1.75 volts per cell). As batteries age, the available capacity decreases. For the same ampere‐hours removed, an aged battery bank will reach a lower voltage compared to the same battery bank when it was new. Using the PVX‐3050T as an example, when the available capacity declines to 50% of its nameplate rating, it will only run 12 hours to the endpoint voltage. Now take a scenario where the LVD is set at a voltage that corresponds to 50% DOD. The new battery bank will not normally hit this voltage setpoint, but an aged battery bank has less available capacity and may reach the LVD more frequently and the generator will turn on. Thus, the reason for the generator running more frequently is that the battery bank has lost some of its capacity. Note that sometimes a conditioning charge can restore some or all of the lost capacity. Instructions for doing a conditioning charge are given in the Technical Manual.
How do Sun Xtender® batteries compare with Lithium‐Ion batteries?
Sun Xtender® batteries have been successfully employed in energy storage applications for over 30 years and are still gaining in popularity. Lithium‐ion batteries, initially developed for portable power applications such as laptop computers and cells phones, are starting to be introduced for energy storage applications. So, it is natural to ask, which type of battery should I select for my energy storage system? To answer to this question, there are three main aspects to consider:
- Life Cycle Cost
- Size and Weight
- Risks
Life Cycle Cost
The life cycle cost can be defined as the up‐front cost of the batteries divided by the cumulative capacity delivered. The cumulative capacity is the Ah per cycle times the total number of cycles the battery can deliver. In general, the up‐front cost of Lithium‐Ion batteries is 3‐5 times more than Sun Xtender® batteries for the same voltage and capacity rating. To offset this higher up‐ front cost, Lithium‐ion batteries are expected to last longer. Estimates for cycle life vs. DOD vary widely, but the cumulative capacity generally works out to be 3‐5 times more than Sun Xtender® batteries. Thus, the life cycle cost (up‐front cost divided by cumulative capacity) is essentially the same for both technologies.
Size and Weight
Lithium‐Ion batteries have a higher specific energy (Wh/kg) and energy density (Wh/L) compared to Sun Xtender® batteries. Although the exact numbers depend on the cell chemistry and the battery pack design, in general, the weight and size of a Lithium‐Ion battery bank will be about one half that of a Sun Xtender® battery bank, for the same voltage and capacity rating. In most energy storage applications, the size and weight of the battery bank are not significant factors. However, if there are size and weight constraints, a Lithium‐ion battery would have an advantage.
Risks
Lithium‐Ion batteries definitely pose additional risks compared to Sun Xtender® batteries. Because they have just recently been introduced for energy storage applications, Lithium‐ion battery pack designs are not necessarily optimized and there are compatibility issues with existing charge controllers and inverter designs. All Lithium‐batteries contain an electronic‐ controlled battery management system (BMS). Even though the Lithium‐ion cells have a longer cycle life than Sun Xtender® batteries, the life of the Lithium‐ion battery pack may be curtailed by failure of the BMS. Sun Xtender® batteries do not contain any electronic components that can fail. One further risk associated with Lithium‐ion batteries is the susceptibility to thermal runaway. Unlike Sun Xtender® batteries, lithium‐ion batteries contain a flammable organic electrolyte. If excessive heat develops inside a single cell (e.g., due to manufacturing defect, abuse or abnormal operation), the electrolyte and other materials in the cell can catch on fire. If just one cell experiences thermal runaway, it can spread to adjacent cells and the entire battery can catch on fire, emit hazardous fumes and smoke, or even explode. Although the risk of this occurrence is considered low, there have been numerous noteworthy field failures of lithium‐ ion batteries leading to recalls (e.g., Dell Notebook computers, Samsung Galaxy Note 7 smartphones) and system redesigns (e.g., Boeing 787 battery system), so this risk should not be ignored.
Based on the above discussion, it can be summarized that Sun Xtender® batteries have similar life cycle cost, greater weight and volume, but less operational risk when compared to lithium‐ ion batteries. Therefore, Concorde Battery believes Sun Xtender® batteries will remain the dominant choice for energy storage systems for many years to come.
Where do I find technical information for Sun Xtender® Batteries?
I’m glad you asked! We have loaded the Sun Xtender® website with a wealth of technical information for easy access and navigation (see: www.SunXtender.com). Here you will find detailed product specifications for the full range of battery models, including physical and electrical characteristics, as well as capacity ratings over a wide range of discharge rates (from 1 hour to 120 hours).
The crown jewel is the Sun Xtender® Technical Manual, which provides detailed instructions for battery sizing, installation and servicing. This manual is where you will find our recommendations for optimum charging parameters, specifically, the bulk, absorb and float voltage settings. Another useful Section in the Technical Manual is the Frequently Asked Questions (FAQs), which provides answers to 16 FAQ’s. Finally, Appendix C contains various tables and charts with information like Battery Load Voltage vs DOD, SOC vs OCV, Self‐discharge Characteristics, Discharge curves, Peukert plots, Capacity vs Temperature, Charge Voltage vs Temperature, and Cycle Life vs DOD.
The content & opinions in this article are the author’s and do not necessarily represent the views of AltEnergyMag
Comments (0)
This post does not have any comments. Be the first to leave a comment below.
Featured Product
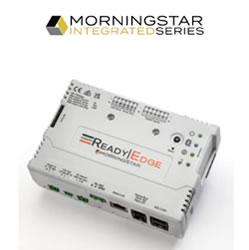