How nanomaterials can trigger a new source of energy
When you want to disgorge a cucumber, you sprinkle it with salt. This creates a very salty solution on its surface that causes water to move from the inside of the cucumber to the outside. This is an example of osmosis. The key point is that the water moves under the action of a driving force, called the osmotic pressure, caused by the concentration difference of salt. This force is also important in our harnessing of blue energy, a sustainable type of power from sea and river water. Here, Stéphane Pleutin, Ph.D, on behalf of materials database Matmatch, explains the process and the potential value of nanomaterials.
Osmotic forces are found in the biological world in a considerable variety of phenomena: to store energy, induce mechanical motion, or control ejection and absorption of compounds, for instance.
Humanity faces a major challenge for the near future: if we want to minimise climate change caused by human activity, it is of the utmost importance to change our energy consumption habits and turn to viable, renewable and non-polluting energy sources.
In this context, nanomaterials play a role that we believe will become essential in the future, providing solutions to technical problems and often opening up new perspectives.
In our search for new energy solutions, it might be useful to draw inspiration from living organisms. For example, research mimicking photosynthesis is being conducted to find new solutions for energy storage.
We are also seeking to use osmotic pressure as a new source of energy in the same way as wind, hydraulic force or the sun. More precisely, we can ask ourselves whether it would be possible to use osmotic force to efficiently extract energy from sea water, the so-called blue energy.
Blue energy is the energy that could be harvested from differences in salinity, by mixing sea water and fresh river water. An estimate could be done by counting estuaries over the Earth, amounting to 8,500 TWh. When we compare this to energy production from other resources, we see the scale of the potential. In 2015, hydraulic energy production was about 4,000 TWh, nuclear energy around 2,600 TWh and wind and solar a combined 1,100 TWh.
According to this estimate, blue energy is so plentiful that it could feed all our needs, if we can just find an effective way to tap it.
How can we manage this fantastic driving force to harvest electricity? Among several approaches that are being developed, the most promising are Pressure-Retarded Osmosis (PRO) and Reverse Electro-Dialysis (RED).
PRO uses the flow of water through the membranes, producing pressurised water that generates electricity using mechanical turbines. RED, on the other hand, uses membranes for ion transport, not water, and the electrical current generated is captured directly from the flow of ions. This technique is currently being explored by REDStack on a large scale with a prototype plant in the Netherlands.
Despite encouraging results, none of the current attempts have yet reached a sufficient power density to be considered as economically profitable.
Both strategies rely on separation of water from ions or ions from water. Yet, the main source of difficulties of these systems is the membrane. To be completely impermeable to the solute molecules, the membrane may only be slightly permeable to the solvent molecules, or vice versa.
Indeed, for the salt not to pass through we need a membrane that has nanometric to sub-nanometric pores. With such small pores, the permeability of the membrane is extremely low and therefore, the water flow is not very intense. So, even if the pressure difference is gigantic, the power that can be recovered from these systems is measured in a few W/m2, below or sometimes slightly above the threshold of economic viability that is estimated to be 5 W/m2.
Membranes with new nanomaterials
For blue energy to become a large-scale exploitable and competitive energy source in the future, it is necessary to make progress not only in the design of membranes, but also in our understanding of the mechanisms at work.
Major efforts have been made in recent years through multidisciplinary research combining chemistry, materials science and nanoscale fluid dynamics. In 2013, membranes made of boron nitride (BN) nanotubes were investigated. These are materials structurally similar to carbon nanotubes, except that carbon atoms are substituted by nitrogen and boron atoms.
Nanotubes with a radius of tens of nanometres showed an osmotic energy conversion density of several kilowatts per square meter, three orders of magnitude larger than the energy conversion density obtained with PRO or RED processes. In 2016, membranes made of an atomically thin molybdenum disulphide (MoS2) layer with nanopores of few nanometres in diameter led to an outstanding power density of 106 W.m-2.
These remarkable performances are in part attributed to the extremely narrow widths of the membranes. Indeed, the flow through the membrane is expected to be inversely proportional to its width. One year later, atomically thin membranes based on hexagonal BN and graphene were investigated showing power densities in the range of 700 W.m-2. All these recent results of extremely high osmotic power densities for devices using new materials as membrane supports have undoubtedly revived the field.
With our recent discoveries we have certainly made great progress on the road to ideal artificial membranes and, in the meantime, have advanced our knowledge, but there is still a long way to go. Perhaps we should listen more carefully to what nature has to tell us about how to effectively harness blue energy.
Matmatch offers a material consultancy service to connect engineers and product developers with materials suppliers and consultants, as well as providing reliable, up-to-date technical information of commercially available materials for energy generation projects on its website.
Featured Product
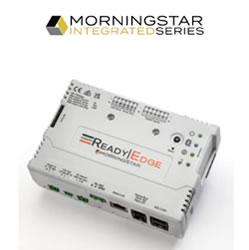