The impending energy crisis and global warming warrant the need for eco-friendly sources of energy. Non-conventional, carbon-neutral sources of energy such as solar, wind, biomass and nuclear energy are being explored and exploited to a considerable extent. At this juncture, when the atmosphere is laden with greenhouse-gases, we can least afford to release stored carbon. The following article gives an account of the basic principles involved in the functioning of Microbial Fuel Cells and the major applications of Microbial Fuel Cell technology, as it stands today.
A. S. Vishwanathan and S. Siva Sankara Sai | Sri Sathya Sai University
The operational and functional advantages of MFCs are:
-
MFCs use organic waste matter as fuels and readily available microbes as catalysts.
-
MFCs do not require highly regulated distribution systems like the ones needed for Hydrogen Fuel Cells.
-
MFCs have high conversion efficiency as compared to Enzymatic Fuel Cells, in harvesting up to 90% of the electrons from the bacterial electron transport system.
Biochemical basis
How do microbes derive nutrition for their sustenance? Chemotrophic microbes utilize organic and other biodegradable compounds, under diverse conditions. The electrons resulting from the oxidation are conveyed to an electron transport chain, across appropriate electron carriers depending on the terminal electron acceptor molecule. In aerobic organisms, this terminal acceptor is oxygen which takes up the electrons and gets reduced to water.
The chemiosmotic hypothesis states that electron transfer chains of bacteria are coupled to the translocation of protons across the membranes which is in turn linked to ATP synthesis by the proton electrochemical potential across the energy tranducing membrane [4].
The bacterial cell membrane functions as an energy transducing membrane operating according to the chemiosmotic principle. The translocation of protons towards the outside of the membrane results in the establishment of a proton electrochemical gradient. The pH gradient adds up to this membrane potential and results in the proton motive force. The re-entry of these protons across the ATP-synthase enzyme is accompanied by ATP synthesis. The ATP synthesised thus is used by the bacteria for their survival [5].
Keeping this theoretical background in mind let us now see how an MFC functions. A typical MFC (see Fig. 1) consists of two compartments - the anodic and cathodic half-cells - which are separated by a selectively permeable, cation-specific membrane or a salt-bridge. The anodic chamber consists of microbes suspended under anaerobic conditions in the anolyte and the cathodic chamber contains the electron acceptor (oxygen). In essence, the electron donor is physically separated from the terminal electron acceptor across the two chambers. Most of the electrons released from the process of oxidation are conveyed to the anode. Electron transfer to the anode can be accomplished by electron mediators or shuttling agents [6], directly by the cell [7] or by means of ‘nanowires’ [8]. These electrons are directed to the cathode across an external circuit and for every electron conducted, a proton is transported across the membrane to the cathode for completing the reaction and sustaining the electric current [9].
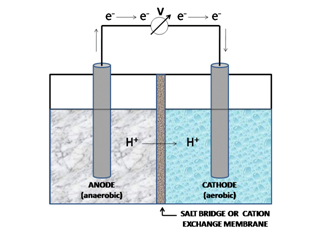
It must however be noted at this juncture, that MFCs can be constructed in different shapes and designs, a description of which is beyond the scope of this article.
Applications of microbial fuel cell technology
Although MFCs have been studied as an alternative energy source, their application is presently limited to certain niche areas. With further improvements in design, cost effectiveness and performance efficiency based on these near-term applications, it would be possible to scale-up and use MFCs as a renewable energy resource.
Micro-organisms can perform the dual duty of degrading effluents and generating power. MFCs are presently under serious consideration as devices to produce electrical power in the course of treatment of industrial, agricultural, and municipal wastewater. When micro-organisms oxidize organic compounds present in waste water, electrons are released yielding a steady source of electrical current. If power generation in these systems can be increased, MFCs may provide a new method to offset operating costs of waste water treatment plants, making advanced waste water treatment more affordable in both developing and industrialized nations [10]. In addition, MFCs are also known to generate less excess sludge as compared to the aerobic treatment process [11].
Powering underwater monitoring devices
Data on the natural environment can be helpful in understanding and modeling ecosystem responses, but sensors distributed in the natural environment require power for operation. MFCs can possibly be used to power such devices, particularly in river and deep-water environments where it is difficult to routinely access the system to replace batteries. Sediment fuel cells are being developed to monitor environmental systems such as creeks, rivers, and oceans [12]. Power densities are low in sediment fuel cells because of both the low organic matter concentrations and their high intrinsic internal resistance. However, the low power density can be offset by energy storage systems that release data in bursts to central sensors [13].
Power supply to remote sensors
With the development of micro-electronics and related disciplines the power requirement for electronic devices has drastically reduced. Typically, batteries are used to power chemical sensors and telemetry systems, but in some applications replacing batteries on a regular basis can be costly, time-consuming, and impractical. A possible solution to this problem is to use self-renewable power supplies, such as MFCs, which can operate for a long time using local resources. Extensive research toward developing reliable MFCs to this effect, is focused mostly on selecting suitable organic and inorganic substances that could be used as sources of energy [14].
BOD sensing
Another potential application of the MFC technology is to use it as a sensor for pollutant analysis and in situ process monitoring and control. Biological Oxygen Demand (BOD) is the amount of dissolved oxygen required to meet the metabolic needs of aerobic organisms in water rich in organic matter, such as sewage. The proportional correlation between the coulombic yield of MFCs and the concentration of assimilable organic contaminants in wastewater make MFCs possible usable as BOD sensors. An MFC-type BOD sensor can be kept operational for over 5 years without extra maintenance, far longer in service life span than other types of BOD sensors reported in the literature [3].
Hydrogen production
Hydrogen production by modified MFCs operating on organic waste may be an interesting alternative. In such devices, anaerobic conditions are maintained in the cathode chamber and additional voltage of around 0.25 V is applied to the cathode. Under such conditions, protons are reduced to hydrogen on the cathode. Such modified MFCs are termed bio-electrochemically assisted microbial reactors (BEAMR) [15].
Conclusion
Though this technology is quite promising as a source of renewable energy, it will be some time before large-scale, highly efficient MFCs enter the commercial scene. The different research groups working around the world will definitely overcome the shortcomings being faced today, enthused and motivated by the immediate need for alternate energy.
References
-
Potter, M. (1911). Electrical effects accompanying the decomposition of organic compounds. Proceedings of the Royal Society of London. Series B, Containing Papers of a Biological Character (1905-1934), 84(571), 260-276.
-
Du, Z., Li, H. and Gu, T. (2007). A state of the art review on microbial fuel cells: A promising technology for wastewater treatment and bioenergy. Biotechnology Advances, 25, 464-482.
-
Lovley, D. R. (2006). Bug juice: Harvesting electricity with microorganisms. Nature Reviews Microbiology, 4, 497-508.
-
Nicholls, D. G. (1982). Bioenergetics – An Introduction to the chemiosmotic theory. London: Academic Press.
-
Moat, A. G., Foster, J. W. and Spector, M. P. (2002). Microbial Physiology (4th ed.). New York: John Wiley and Sons.
-
Rabaey, K., Boon, N., Siciliano, D., Verhaege, M. and Verstraete, W. (2004). Biofuel cells select for microbial consortia that self-mediate electron transfer. Applied and Environmental Microbiology, 70(9), 5373-5382.
-
Bond, D. R. and Lovley, D. R. (2003). Electricity production by Geobacter sulfurreducens attached to electrodes. Environmental Science and Technology, 69(3), 1548–1555.
-
Reguera, G., Nevin, K. P., Nicoll, J. S., Covalla, S. F., Woodard, T. L. and Lovley, D. R. (2006). Biofilm and nanowire production leads to increased current in Geobacter sulfurreducens fuel cells. Applied and Environmental Microbiology, 72(11), 7345-7348.
-
Logan, B. E. and Regan, J. M. (2006). Electricity-producing bacterial communities in microbial fuel cells. Trends in Microbiology, 14(12), 512-518.
-
Shukla, A., Suresh, P., Berchmans, S. and Rajendran, A. (2004). Biological fuel cells and their applications. Current Science 87, 455-468.
-
Kim, B. H., Chang, I. S. and Gadd, G. M. (2007) Challenges in microbial fuel cell development and operation. Applied Microbiology and Botechnology 76, 485-494.
-
Bond, D. R., Holmes, D. E., Tender, L. M. and Lovley, D. R. (2002). Electrode-reducing microorganisms that harvest energy from marine sediments. Science, 295, 483-485.
-
Logan, B. E., Hamelers, B., Rozendal, R., Schröder, U., Keller, J., Freguia, S., Aelterman, P., Verstraete, W. and Rabaey, K. (2006). Microbial fuel cells: Methodology and technology. Environmental Science and Technology, 40(17), 5181-5192.
-
Shantaram, A., Beyenal, H., Veluchamy, R. and Lewandowski, Z. (2005). Wireless sensors powered by microbial fuel cells. Environmental Science and Technology 39, 5037-5042.
-
Liu, H., Grot, S. and Logan, B. E. (2005) Electrochemically assisted microbial production of hydrogen from acetate. Environmental Science and Technology 39, 4317-4320.
The content & opinions in this article are the author’s and do not necessarily represent the views of AltEnergyMag
Comments (0)
This post does not have any comments. Be the first to leave a comment below.
Featured Product
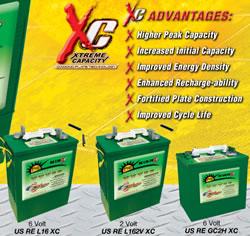