Solar energy has a large potential as a renewable desalination energy source because of its abundance on Earth. Furthermore, advances in solar-wind hybrid desalination systems will be looked at, that demonstrate high efficiency and low carbon emissions as well.
Advancements in Desalination Using Solar Energy
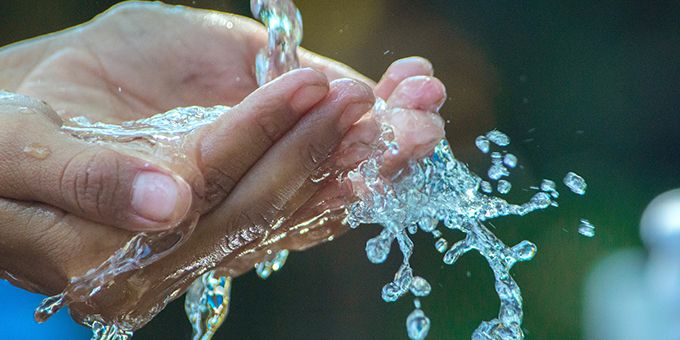
Dr. Raj Shah and Amanda Loo | Koehler Instrument Company
Introduction
Freshwater is essential for people around the world. However, with the scarcity of freshwater that we are currently encountering, desalination processes need to be improved to efficiently generate clean and safe water. A major issue with desalination systems is that they are very energy-intensive and release harmful emissions into the environment when using fossil fuels. Switching to using renewable sources such as solar energy for desalination systems gives a more environmentally friendly approach to desalination and helps to combat water scarcity.
Advancements in solar energy desalination processes, including electrodialysis and reverse osmosis, will be looked at in this paper. Solar energy has a large potential as a renewable desalination energy source because of its abundance on Earth. Furthermore, advances in solar-wind hybrid desalination systems will be looked at, that demonstrate high efficiency and low carbon emissions as well.
Water Scarcity
Water is an essential resource to people and other lifeforms. Freshwater is used for nourishment, cleaning, and agriculture throughout the world. 97% of Earth’s water is saline and only 3% is freshwater that can be used for life processes, making it essential that desalination processes are properly performed and well developed. Occasional, temporary, or perpetual droughts can cause shortages in freshwater supply [1]. Population increase and industrialization are also contributing to the decreasing water supply that is presently being observed [2].
Figure 1. Projected global water stress in 2040 [3].
Water withdrawal refers to the act of removing water from available resources, such as rivers or lakes, with some of this withdrawn water returning to the original sourcing through leakage or other ways [4]. As Figure 1 depicts, countries like the US, China, India, and Australia are expected to have high ratios of withdrawal to supply, while northern Africa and western Asia demonstrate extremely high ratios. Therefore, there must be an adequate freshwater supply for people to use as the world demand remains high.
There are several different methods to measure water scarcity. One of these methods is the Falkenmark Indication, which looks at the number of people in an area and the volume of available water. According to this method, a volume of 1700m3/cap/year of available renewable freshwater per person means that the given area is experiencing water scarcity. A value of 1000m3/cap/year demonstrates high water scarcity, and 500m3/cap/year demonstrates absolute scarcity. The water use to availability ratio can also be used, where the available renewable water resources and amount of water used are looked at [4]. With these different standards to determine water scarcity, there are also two different types of water scarcity, physical and economic [1]. Physical water scarcity is associated with limited access to water resources, with the Middle East and North Africa (MENA) region, southeast Europe, Western Australia, and India being areas experiencing physical water scarcity. Economic water scarcity is the inability to have access to available water resources due to institutional, political influences, or other contributing factors. Central and Latin America, South and Central Africa and Asia regions demonstrate economic water scarcity.
Solar Energy Desalination
There has been an observed correlation that areas with high solar radiation intensity are typically in high need of a clean water supply [5]. There is an expected 9% desalination market growth worldwide in the years 2018-2022, with 74% of this growth mainly from Europe and the MENA region. Solar energy is a promising source for desalination because 70% of the sun’s radiation can be harvested and is a permanently available source [3]. Figure 2 demonstrates different pathways that solar energy can be harvested with and the resulting applications it can be used.
Figure 2. Solar energy harvesting and purposes [6].
With the various methods of harvesting and the availability of solar iridescence, there is a promising outlook for solar energy use for desalination. There are two current types of solar energy-based desalination systems, direct and indirect. Direct systems are when distillate is produced in the solar collector, while indirect systems are when solar energy is harvested and used for desalination later. An example of a direct system is solar stills, where solar radiation is absorbed and directly transferred to the saline water input, resulting in the water evaporating and being collected while the salts and particles are drained [7]. This system has low cost but low performance, making it better for isolated areas without a high-water demand. Nanomaterials like carbon, graphite, nanoplatelets, and silicon dioxide can be used to make direct solar desalination more efficient.
Indirect systems include membrane distillation, reverse osmosis (RO), and electrodialysis (ED) [3]. Membrane distillation has high resulting water purity and can be used with renewable energy but has a higher energy consumption when compared to RO and other desalination procedures [2]. Reverse osmosis is by far the most popular desalination method, with 65% of the world’s desalinated water being obtained from this method [8].
Electrodialysis
9% of solar energy desalination technologies in 2011 were from electrodialysis. A standard ED system requires between 6-11kW/m3, but this value is largely dependent on the feed water [9]. Ion exchange membranes are used in ED systems and result in high water recovery. Using these membranes means that reactions or chemicals are not required to produce freshwater, demonstrating environmental friendliness. Furthermore, ED systems have high water recovery rates and their membranes have long lifetimes [10]. Different feed waters can be used for desalination purposes with different levels of salinity.
Table 1. Water classes based on electrical conductivity (EC) and total dissolved solids (TDS).
Table 1 shows different classifications of feed waters, with safe drinking water having EC <0.7mS/cm and TDS <0.5g/L. In a 2017 study, a brackish water electrodialysis desalination system was designed with 10L of 0.5M Na2SO4 as an electrolyte and feed water from Northern Chile categorized as moderately saline [9]. The pretreatment consisted of column filtration, with column 1 composing of a multimedia filter and column 2 composing of an ion-exchange softener. Columns 3 and 4 both consisted of equal values of granular ferric hydroxide for arsenic removal. A photovoltaic system was used as a power source with a 19° angle and two LiFePO4 batteries used as electricity storers from the PV panels. Four different trials were conducted, and results showed that the pretreatment of arsenic removal did not affect desalination time when there is a direct connection to batteries. Overall, a shorter desalination time was observed when there was a direct connection to batteries. 95.6-98.2% TDS were removed in this designed electrodialysis system. However, >93% nitrate was removed because of high initial concentrations in the feed water, meaning that particle removal depends on initial concentration.
Electrodialysis with bipolar membranes (EDBM) can produce HCl and NaOH from desalination with waste electricity by removing ionic and non-ionic components [11, 10]. Using variable current intensities to emulate PV modules as a power supply and synthetic seawater desalination brines, ways to reduce energy consumption can be determined. Four different systems were looked at, with a standard system undergoing constant intensity mode with a continuous operation mode. System 1 and system 2 had continuous operation modes with variable current and no feedback control loop. System 1 had 0.5M NaOH as the electrode and system 2 had 0.25M Na2SO4 as the electrode. Finally, system 3 had a semi-continuous operation mode with a feedback central loop. The specific energy consumption (SEC) of HCl production was looked at, which was determined by the EDBM stack production of HCl from brine solutions. The results show that system 3 had the lowest SEC, with a value of 4.4kW/kg while the standard system had 7.3kW/kg HCl production. The presence of a feedback loop and the semi-continuous operation mode of system 3 demonstrated reduced energy consumption with its improved SEC HCl production.
Reverse Osmosis
Reverse osmosis has been widely used as a main source of desalination. Advantages of RO systems compared to other desalination methods are that they can be used at a small or large scale and have low CO2 emissions [5]. For different amounts of treated water, there are different power consumption values, with the greater flow rate corresponding to higher power consumption [12]. The energy consumption of RO systems is a major concern because they require a significantly greater amount of energy compared to other desalination systems [13]. Table 2 shows different specifications of commercial RO systems. All the systems have permeated and raw water TDS of <500mg/L and <5000mg/L, respectively. A RO system with a 50m3/day permeate flow rate has the least required power consumption of 4.1kW while a RO system with a 1000m3/day permeate flow rate that has the greatest power consumption of 52kW.
Table 2. Commercial RO unit specifications with low energy requirements [12].
Because renewable energy has been a popular topic in recent years, many studies have been done on renewable energy reverse osmosis desalination systems to combat water scarcity. Solar and wind energy have been the most researched renewable energies for desalination because of their natural abundance in the world. 43% of renewable-powered desalination plants are solar PV, and 27% are solar thermal [7], meaning that many advancements have been made in solar energy photovoltaic reverse osmosis systems to increase water output efficiency and lower costs.
Photovoltaic Reverse Osmosis
Photovoltaic systems for reverse osmosis (PV-RO) plants are cheaper than solar thermal plants, as seen by the price change of $33.44/watt in 1979 to $0.35/watt in 2017 for photovoltaic modules [8, 5]. Photovoltaic modules use solar energy that is oftentimes readily available, and in doing so, reduces the carbon footprint. The PV modules convert electrical power from solar radiation to be used in the RO process [14].
Figure 3. PV-RO desalination plant set-up with dashed lines representing optional components [15].
Figure 3 shows a standard PV-RO desalination plant with common steps and materials used. PV modules in a PV-RO system are typically made of mono-crystalline or multi-crystalline silicon. Installing an automatic tracking system that moves during the day according to the sun’s hourly positions can increase power output compared to using flat modules. However, this advancement is expensive, so maximum power point tracker (MPPT) circuits can be used for the same purpose but more cost-effectively. A pretreatment process involves filtering particles from the saline feed water, such as free chlorine by active carbon filtration. Batteries allow the system to operate during the night or when there is not a lot of sun radiance available, meaning that PV-RO plants can continuously run. A disadvantage of batteries is that they can reduce efficiency and increase system complexity. Advancements have been made in PV-RO plants to remove the need for batteries and in place use other devices for energy storage.
Fuel cells can be used for electricity conversion from chemical energy in place of batteries. Batteries are more expensive and less efficient in energy storage compared to fuel cells. Using proton exchange membrane fuel cells (PEMFCs), hydrogen is used to produce electricity with water produced as a byproduct. PEMFCs have no environmental impact and can be used with other devices such as wind turbines to generate electricity. The greater energy storage efficiency is fitting for use in place of batteries for RO systems [12].
Rankine cycle reverse osmosis systems (RC-RO) are also popular and are composed of a solar field, Rankine cycle power plant, and RO unit [8]. The solar field thermal energy is used on the RC working fluid to evaporate it, and the fluid is then used for the turbines to generate power. This generated power is then used in the RO system high-pressure pump to begin the reverse osmosis desalination process. It has been determined that when using water as a working fluid, as plant production capacity increases, the price of resulting desalinated water per m3 decreases. In organic Rankine cycle reverse osmosis systems (ORC-RO), organic working fluids are used, such as hydrocarbons and fluorocarbons, allowing for use in remote areas. An advancement experimentally determined for ORC-RO systems is using a double-cascade of ORC [14]. Using these materials in a two-cycle system reduces the amount of required space and increases performance efficiency. The cycles consisted of a top cycle that generated energy for the RO high-pressure pump and a bottom cycle that provides energy for other components by using the thermal energy waste of the top cycle.
Hybrid Wind and Solar RO Desalination
A diesel generator in hybrid systems acts as a “backup power source” to ensure efficient energy production. In a 2018 study in Turkey, reverse osmosis desalination systems were studied with all of them being able to provide fresh water for 1200 people daily, with a value of 20L of freshwater/day per person. Photovoltaics, wind turbines, and diesel generators were looked at as potential RO system components. Using ROSA software, a RO design program that looks at the flow rate, energy consumption, and other important values, and HOMER, an optimization tool that analyzes techno-economic values of PV panels, turbines, batteries, etc., the most efficient and economical RO system was determined.
Table 2. HOMER analysis of seven different RO cases (Case 1, diesel system; Case 2, wind system; Case 3, PV system; Case 4, PV-wind; Case 5, PV-diesel; Case 6, wind-diesel; Case 7, PV-wind-diesel hybrid system) [13].
According to Table 2, Case 4 had 58.33% electricity generated by the solar panels and 41.67% generated by the wind turbines. Case 6 had 77.38% electricity generated from wind turbines and 22.62% generated by the diesel generator. Using a diesel generator will inevitably release pollutants into the environment when burning diesel fuel, such as CO2, CO, and SO2, so the pollutant emissions of Cases 1, 5, 6, and 7 were looked at. Case 7 had the least number of emissions. Also, the levelized cost of water (LCOW) was looked at for each case, and again Case 7 had the lowest value of $2.20/m3, with the standard price of diesel fuel used being $1.25 in Bozcaada, Turkey. Overall, the hybrid Case 7 can be concluded as the best option out of the other cases for its low LCOW and low emission characteristics. Case 2 and case 3 demonstrated significant electricity generation potential, with values of 286,641kW and 184,881kW, respectively.
Solar chimneys are another innovation to the reverse osmosis process. They are designed to use incident solar radiation to heat the air inside of a tower. The air is then directed to the center of the tower to be used in wind turbines, which in turn generate electricity [7]. An example of a solar chimney’s design is shown in Figure 4.
Figure 4. Typical solar chimney design.
A major shortcoming of PV-RO systems is that PV power output decreases with the accumulation of sand and dust, meaning that arid regions are not ideal for PV-RO systems. High temperatures can also reduce PV conversion efficiency, further demonstrating how hot and arid regions are undesirable. Solar radiance is found to be the most important factor in PV-RO efficiency, with the optimal radiation range for PV-RO systems being 400-1200W/m2 [14]. Solar towers are ideal solutions to these problems for PV-RO systems because they do not need constant solar radiation as the ground acts as a heat storage system. They also do not use exposed surfaces such as mirrors or lenses, so sand and dust do not pose a significant threat.
Solar chimneys are known to have low production costs but low thermal efficiency as well. Solar chimney power plants (SCPP) consist of a solar chimney, collector, and turbine. A cooling tower (CT) also consists of a solar chimney and collector but does not have a turbine. In a 2020 experiment, a hybrid solar chimney power plant (HSCPP) was created by combining an SCPP and CT [16]. The results of combining these two systems were that electricity and distilled water were produced for 24 hours because CTs can operate at night and without the presence of the sun. A 40% increase in electrical power production in kW/year was observed compared to a standard SCPP and a 40% higher CO2 emissions reduction in the hybrid system.
Conclusion
Solar energy desalination systems have undergone many advancements that have increased water output and decreased energy consumption. In electrodialysis, the use of feedback loops allowed for better HCl acid production according to its specific energy consumption. Also, a direct connection to batteries resulted in a quicker desalination process, meaning that more freshwater could be produced in the same amount of time. Both of these advancements showed reduced energy consumption. Using a double cascade of organic Rankine reverse osmosis cycles makes this desalination process more efficient and reduces the space needed. Solar chimneys can be used with wind turbines to generate more electricity for reverse osmosis processes. RO is the most promising desalination method for renewable sources, as the greatest number of advancements have been made for this process. It is expected that as the world population increases and water becomes more scarce, further advancements in desalination methods will improve to keep up with the growing freshwater demand.
The authors are grateful to Mr. Nathan Aragon for his review of this manuscript.
About Dr. Raj Shah
Dr. Raj Shah is a Director at Koehler Instrument Company in New York, where he has worked for the last 25 years. He is an elected Fellow by his peers at IChemE, CMI, STLE, AIC, NLGI, INSTMC, The Energy Institute and The Royal Society of Chemistry. An ASTM Eagle award recipient, Dr. Shah recently coedited the bestseller, “Fuels and Lubricants handbook”, details of which are available at https://www.astm.org/DIGITAL_LIBRARY/MNL/SOURCE_PAGES/MNL37-2ND_foreword.pdf
About Amanda Loo
Ms. Amanda Loo is a student of Chemical engineering at SUNY, Stony Brook University, where Dr. Shah is an adjunct professor and the chair of the external advisory Committee in the Dept. of Material Science and Chemical Engineering.
References
[1] Gude, V. G., “Desalination and water reuse to address global water scarcity,” Reviews in Environmental Science and Bio/Technology, 16(4): 591-609, 2017
[2] Miladi, R., Frikha, N., Kheiri, A., & Gabsi, S., “Energetic performance analysis of seawater desalination with a solar membrane distillation,” Energy Conversion and Management, 185: 143-154, 2019, https://doi.org/10.1016/j.enconman.2019.02.011
[3] Ahmed, F. E., Hashaikeh, R., & Hilal, N., “Solar powered desalination–Technology, energy and future outlook,” Desalination, 453: 54-76, 2019, https://doi.rg/10.1016/j.desal.2018.12.002
[4] Liu, Junguo, et al., "Water scarcity assessments in the past, present, and future," Earth's future, 5(6): 545-559, 2017, doi:10.1002/2016EF000518
[5] Mito, M. T., Ma, X., Albuflasa, H., & Davies, P. A., “Reverse osmosis (RO) membrane desalination driven by wind and solar photovoltaic (PV) energy: state of the art and challenges for large-scale implementation,” Renewable and Sustainable Energy Reviews, 112: 669-685, 2019, https://doi.org.10.1016/j.rser.2019.06.008
[6] A. Labouret, et al., “Solar Photovoltaics,” Institution of Engineering & Technology, Stevenage, United Kingdom (2010)
[7] Maia, C. B., Silva, F. V., Oliveira, V. L., & Kazmerski, L. L., “An overview of the use of solar chimneys for desalination,” Solar Energy, 183: 83-95, 2019, https://doi.org/10.1016/j.solener.2019.03.007
[8] Shalaby, S. M., “Reverse osmosis desalination powered by photovoltaic and solar Rankine cycle power systems: A review,” Renewable and Sustainable Energy Reviews, 73: 789-797, 2017, https://doi.org/10.1016/j.rser.2017.01.170
[9] Gonzalez, A., Grágeda, M., & Ushak, S., “Assessment of pilot-scale water purification module with electrodialysis technology and solar energy,” Applied Energy, 206: 1643-1652, 2017, https://doi.org/10.1016/j.apenergy.2017.09.101
[10] Al-Amshawee, S., Yunus, M. Y. B. M., Azoddein, A. A. M., Hassell, D. G., Dakhil, I. H., & Hasan, H. A., “Electrodialysis desalination for water and wastewater: A review,” Chemical Engineering Journal, 380: 122231, 2020, https://doi.org/10.1016/j.cej.2019.122231
[11] Herrero-Gonzalez, M., Diaz-Guridi, P., Dominguez-Ramos, A., Ibañez, R., & Irabien, A., “Photovoltaic solar electrodialysis with bipolar membranes,” Desalination, 433: 155-163, 2018, https://doi.org/10.1016/j.desal.2018.01.015
[12] Rezk, H., Sayed, E. T., Al-Dhaifallah, M., Obaid, M., Abou Hashema, M., Abdelkareem, M. A., & Olabi, A. G., “Fuel cell as an effective energy storage in reverse osmosis desalination plant powered by photovoltaic system,” Energy, 175: 423-433, 2019, https://doi.org/10.1016/j.energy.2019.02.167
[13] Gökçek, M., “Integration of hybrid power (wind-photovoltaic-diesel-battery) and seawater reverse osmosis systems for small-scale desalination applications,” Desalination, 435: 210-220, 2018, https://doi.org/10.1016/j.desal.2017.07.006
[14] Kasaeian, A., Rajaee, F., & Yan, W. M., “Osmotic desalination by solar energy: A critical review,” Renewable Energy, 134: 1473-1490, 2019, https://doi.org/10.1016/j.renene.2018.09.038
[15] Ghermandi, A., & Messalem, R., “Solar-driven desalination with reverse osmosis: the state of the art,” Desalination and water treatment, 7(1-3): 285-296, 2009
[16] Abdelsalam, E., Kafiah, F., Tawalbeh, M., Almomani, F., Azzam, A., Alzoubi, I., & Alkasrawi, M., “Performance analysis of hybrid solar chimney–power plant for power production and seawater desalination: A sustainable approach,” International Journal of Energy Research, p. 1-15, 2020, doi:10.1002/er.6004
The content & opinions in this article are the author’s and do not necessarily represent the views of AltEnergyMag
Comments (0)
This post does not have any comments. Be the first to leave a comment below.
Featured Product
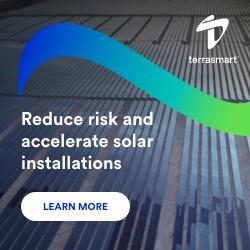